Make your microcontroller apps safe and secure with Rust
Rust on a Device
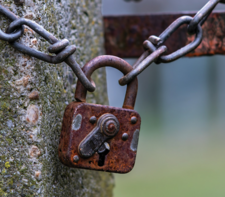
© Photo by Lucas van Oort on Unsplash
Rust, a potential successor to C/C++, claims to solve some memory safety issues while maintaining high performance. We look at Rust on embedded systems, where memory safety, concurrency, and security are equally important.
For decades, C and increasingly C++ have been the languages of choice for microcontroller development, with assembler reserved for optimizations and start-up code. Most vendors provide free development IDEs, and most of these use GNU compilers under the hood. Some of the IDEs come with initialization code and generate device configuration code (choosing I/O functionality, etc.), so a device can be configured in a point-and-shoot manner letting you concentrate on writing application code. Similarly, manufacturers of I/O devices such as sensors and wireless modules supply drivers and example code.
C/C++ is an easy-to-learn language, but it's also easy to abuse and to write buggy code. Embedded code is expected to run unattended, often in hostile environments where code updates are difficult if not impossible. Increasingly, these devices are Internet-connected. While this means easier code updates, it requires a significant amount of extra effort to do well, and ironically this very connectivity lays the device open to nefarious access attempts. I know that there are tools available that help to minimize the risk of bad code getting into a product, and it is certainly not easy to consider rewriting large legacy codebases in another language, but I think that Rust [1] has come far enough and has sufficient advantages over C/C++ to be considered for new code and for re-writing critical sections of existing code as a stepping stone to a full Rust implementation.
With that being said, this article is intended as a "getting started" guide: Adoption of Rust is a big step in a commercial environment, and one of the best ways to evaluate a new language is to try it! In today's world of low-cost development boards and free software tools, all it takes is a little time and determination. Then you can base your decisions on some real-life experience.
[...]
Buy this article as PDF
(incl. VAT)
Buy Linux Magazine
Subscribe to our Linux Newsletters
Find Linux and Open Source Jobs
Subscribe to our ADMIN Newsletters
Support Our Work
Linux Magazine content is made possible with support from readers like you. Please consider contributing when you’ve found an article to be beneficial.
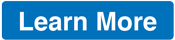
News
-
TUXEDO has unveiled a new InfinityBook Pro with an AMD Ryzen AI 300
This new notebook offers serious power that is ready for your business, development, or entertainment needs.
-
Is This the Year of Linux?
Another major organization has decided to kick Windows and Office to the curb, in favor of Linux.
-
Linux Mint 20 Reaches EOL
With Linux Mint 20 at its end of life, the time has arrived to upgrade to Linux Mint 22.
-
TuxCare Announces Support for AlmaLinux 9.2
Thanks to TuxCare, AlmaLinux 9.2 (and soon version 9.6) now enjoys years of ongoing patching and compliance.
-
Go-Based Botnet Attacking IoT Devices
Using an SSH credential brute-force attack, the Go-based PumaBot is exploiting IoT devices everywhere.
-
Plasma 6.5 Promises Better Memory Optimization
With the stable Plasma 6.4 on the horizon, KDE has a few new tricks up its sleeve for Plasma 6.5.
-
KaOS 2025.05 Officially Qt5 Free
If you're a fan of independent Linux distributions, the team behind KaOS is proud to announce the latest iteration that includes kernel 6.14 and KDE's Plasma 6.3.5.
-
Linux Kernel 6.15 Now Available
The latest Linux kernel is now available with several new features/improvements and the usual bug fixes.
-
Microsoft Makes Surprising WSL Announcement
In a move that might surprise some users, Microsoft has made Windows Subsystem for Linux open source.
-
Red Hat Releases RHEL 10 Early
Red Hat quietly rolled out the official release of RHEL 10.0 a bit early.